Satellite Technology In 2025 And Beyond: The Future of GEO – Avenga
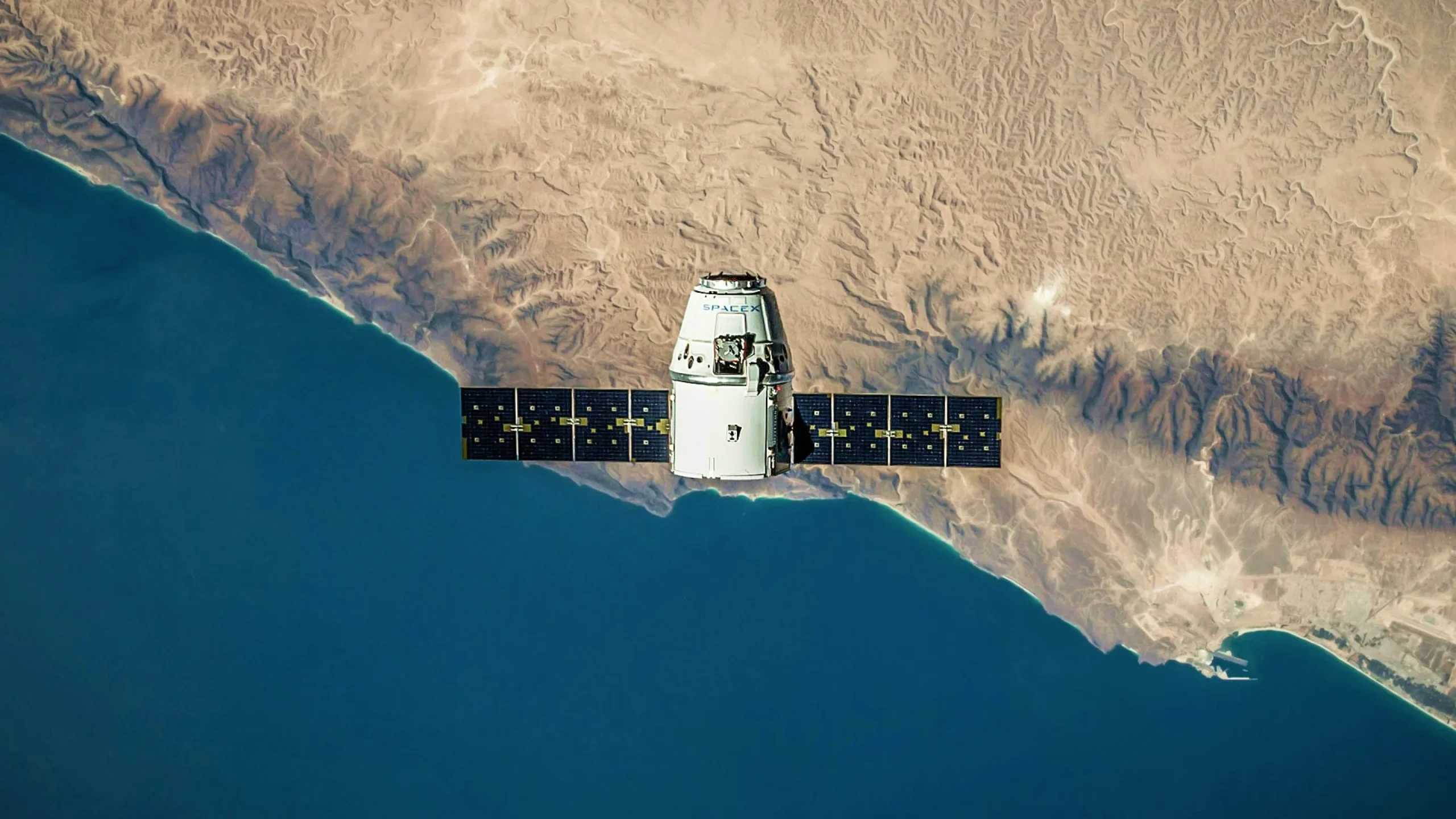
May 12, 2025 9 min read
In recent years, humanity has tremendously evolved. Technologies like artificial intelligence have moved beyond science fiction—they’re now woven into nearly every aspect of our daily lives. Yet the issue of orbital congestion remains obscure and complex, even considering all the latest agile advancements humanity has developed and utilized. But the narrative is evolving.
From the days of large geostationary satellites transmitting television signals across continents, satellite communications have advanced significantly. What started as specialized technology used in defence and television has developed into a crucial component of global infrastructure. Long linked to broadcasting and specialized research applications, satellite industry innovation has made major operators pivotal participants in the face of the world’s most urgent problems. Cloud services, IoT devices, and remote working have all contributed to the rapid growth of digital demands, highlighting the shortcomings of ground-based networks—particularly in underserved or difficult-to-reach areas. In 2023, the satellite services business generated over 110 billion dollars. The growing satellite internet sector has fueled a sharp rise in the number of satellites in Earth’s orbit as demand for anytime, anywhere connection continues to rise.
At the forefront of this change is the emergence of non-terrestrial networks (NTNs), known as networks that completely circumvent conventional ground infrastructure. These days, satellite organizations supply high-speed access to even the most remote and underserved regions of the world by providing connectivity from space. But there’s no need to show our full hand just yet. Keep reading to learn more about how satellite development and other trends are shaping the future of the space industry.
Understanding Non-Terrestrial Networks (NTNs)
Non-terrestrial networks are a revolutionary departure from the traditional view of connection. NTNs employ satellites that orbit the world to deliver coverage straight from the sky, whereas traditional systems have long relied largely on cable and towers on the ground. As a result, there is now the possibility of delivering high-speed, low-latency internet and data services in locations that are far beyond the reach of terrestrial infrastructure, including open waterways, rural villages, mountains, and disaster areas. Expanded coverage is only one aspect of NTNs’ expansion; another is its vision for the future of international communication. Satellite operators are creating reliable, scalable, and borderless systems that eliminate the need for ground infrastructure, enabling everything from national logistics to emergency response.
This graph illustrates that the global NTN market size is gaining momentum. The growth is driven by a number of factors, such as the growing 5G infrastructure, the increasing demand from consumers in urban, suburban areas and remote areas for high-speedlow-latency connectivity, the rise in mobile data traffic, and the growing government initiatives to expand 5G’s customer reach.
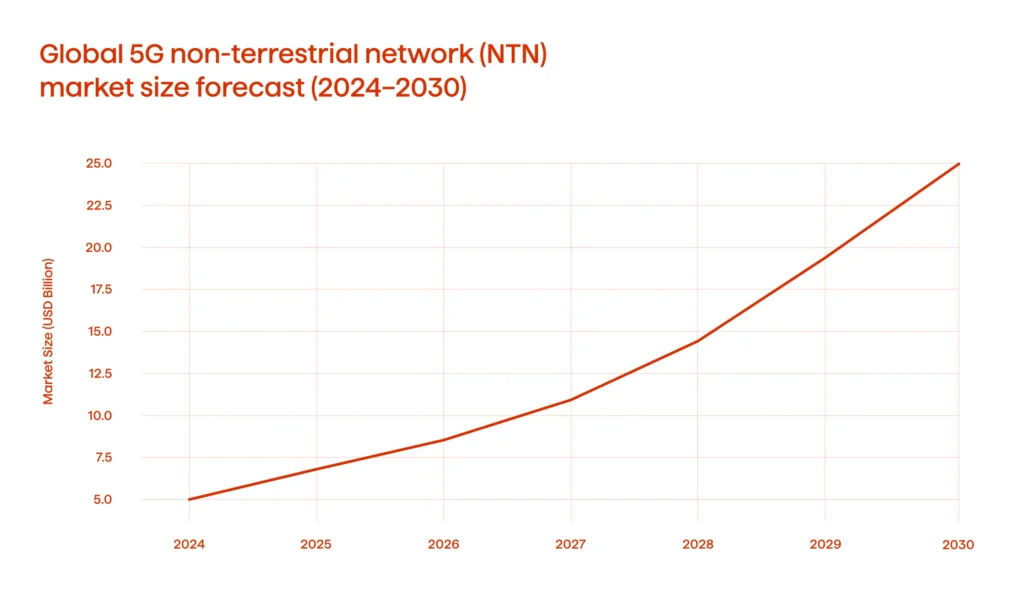
NTNs provide connectivity from above, as opposed to conventional ground-based networks, which necessitate high-density ground infrastructure and are either costly or impractical to deploy in remote or hostile areas. It puts them in a prime position to help underserved communities across all political and geographic borders.
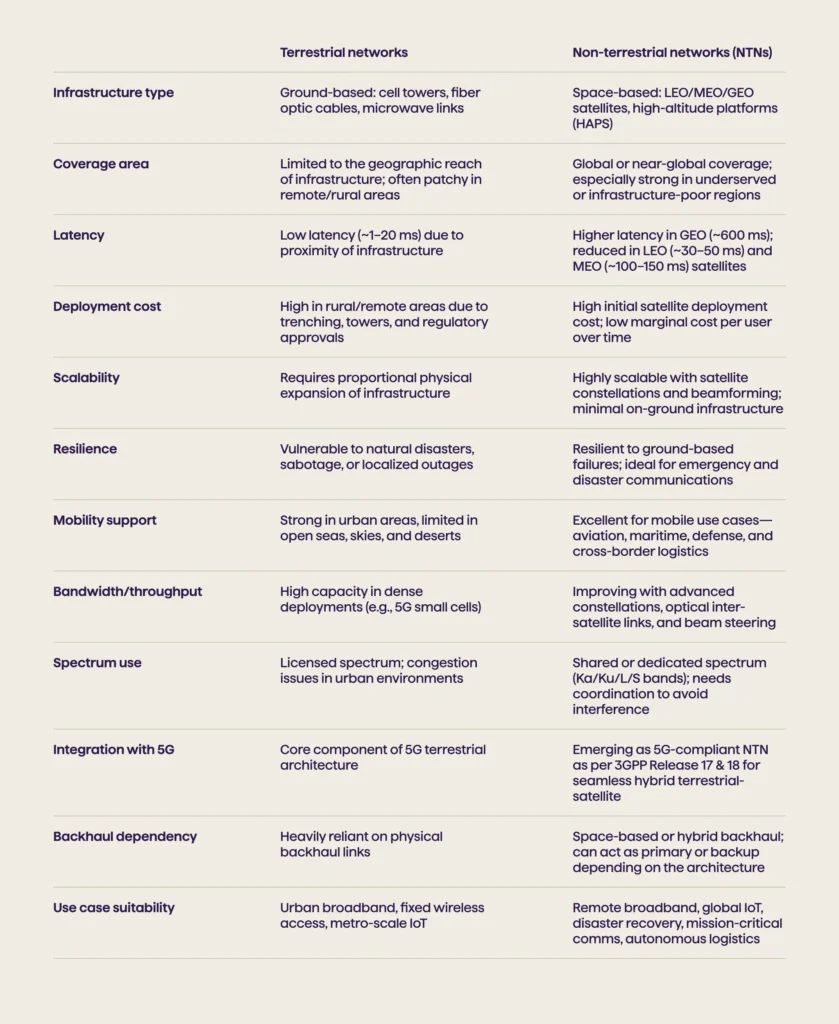
In addition to filling coverage gaps, NTNs are valuable for connecting people and engaging applications at previously unheard-of levels, which highlights their role in achieving broadband expansion, supporting the Internet of Things, and keeping vital communications open during crisis response. This is especially true given the growing demands for real-time data and robust networks. NTNs continue to function even in the event of natural disasters, infrastructure breakdown, or situations where installing physical networks would be logistically or financially impractical due to their independence from terrestrial infrastructure.
The Growing Role of Satellite Constellations
The core of contemporary non-terrestrial networks are the satellite constellations, which are collections of synchronized spacecraft orbiting in shared orbits. To provide worldwide coverage with lower latency and higher throughput, constellations are mostly built on low Earth orbit (LEO) and medium Earth orbit (MEO), as opposed to earlier systems that relied on a small number of massive geostationary (GEO) satellites at an altitude of 35,786 kilometers above the Earth.
LEO constellations, like those offered by Globalstar, OneWeb, Starlink, and Amazon’s Project Kuiper, are made up of hundreds or thousands of tiny satellites in the 500–2,000 km range. Their proximity to Earth’s surface allows for latency as low as 20–40 ms, which is comparable to fiber-based systems on Earth and appropriate for cloud applications, video conferences, and broadband internet. In addition to optical inter-satellite links (ISLs), which allow direct satellite-to-satellite communication and eliminate the need for ground relay stations, these satellites are usually equipped with phased-array antennas and steerable beams for dynamic user link management.
Compared to LEO systems, MEO constellations like O3b and SES offer faster throughput and wider coverage per satellite, though at the expense of somewhat higher latency (~100–150 ms). These are best suited for backhaul solutions in areas without terrestrial infrastructure, enterprise connectivity, and maritime and aviation use cases.
Redundancy and mesh routing features are built into satellite constellations to improve network resilience and reduce single points of failure. Constellations are expanding coverage and propelling the transition to adaptive network designs when paired with AI-based traffic routing and autonomous aircraft operations.
Product Engineering Behind the Satellite Revolution
Modern satellite systems require cutting-edge software, hardware, and system integration product engineering. Miniaturized, lightweight, radiation-hardened components with improved power efficiency and thermal management are essential for satellites, particularly those in low-Earth orbit constellations. When possible, engineers mix specialized RF systems, phased-array antennas, onboard data processors, and COTS components to construct modular designs. Other in-orbit performance enhancers include AI-driven resource allocation and optical inter-satellite links (ISLs).
Ground user terminals must facilitate smooth satellite handoffs, adaptive modulation, and beam tracking. Low-latency signal processing, multi-band antennas, and precision mechanical construction are necessary. To decrease reliance on central nodes and speed up response times, edge computing is being increasingly integrated into space and ground systems.
To verify system integrity, rigorous test beds recreate the severe launch, orbit, and re-entry environments. Delivering scalable, reliable NTN infrastructure that can function within real-world restrictions is the ultimate goal of product engineering.
Ground Devices and Terminals: Bridging User Access
Ground equipment converts signals from orbit into accessible connections, serving as the interface between end users and NTNs. From dynamic mobile terminals in aviation, maritime, and defense to stationary rural deployments, next-generation user terminals are tailored for a broad spectrum of uses, including integration with mobile phones, enabling satellite communication capabilities in everyday devices.
By using electronically steerable phased-array antennas, these terminals can track fast LEO satellites without the need for mechanical components. This feature guarantees uninterrupted communication even when satellites are switched over. Additionally, terminals use adaptive modulation techniques, multi-band support (such as Ka-, Ku-, and S-bands), and sophisticated RF front-ends to provide reliable links in a range of signal and atmospheric conditions.
The majority of ground terminals provide local caching and embedded edge processing to support low-latency data and real-time services, reducing the need for backhaul. Additionally, they offer auto-beam switching and intelligent routing, which are very important in high-interference or mobile scenarios. Handover management, link-layer retransmission, and Doppler shift compensation are very important engineering factors, particularly in mobile contexts. End-user devices must be more compact, power-conscious, and interoperable with terrestrial 5G systems to fully implement hybrid patterns of connectivity as NTNs expand. The ground gear has evolved into intelligent adaptive nodes that complete the satellite loop rather than merely sitting there.
A particularly exciting frontier in this evolution is the integration of solar-powered IoT devices. These self-sustaining devices can harness solar energy to maintain their functionality, ensuring that remote and off-grid applications can operate autonomously, without relying on traditional power sources.
Challenges and the Path Forward
NTNs have advanced quickly, but there are still many operational, legal, and technical obstacles to overcome. Perhaps the most urgent of them is spectrum management. Radio frequency band competition is growing due to the increase in satellite launches, with over 2,800 LEO satellites launched in 2023 alone. To prevent signal interference and guarantee service quality, operators and regulatory organizations such as the ITU must effectively coordinate their spectrum.
Space debris is another growing threat. The chance of a collision increases with the number of satellite constellations, especially in low Earth orbit. According to the latest data, there are over 36,000 objects in orbit that are larger than 10 cm. To ensure long-term sustainability, operators must invest in debris mitigation technology, responsible de-orbiting procedures, and collision avoidance systems.
Another issue is interoperability with ground networks. The performance of a hybrid network requires synchronization of satellite 5G equipment, shared protocols, and seamless handovers. Since the completion of Release 18 and the rollout of its features, attention has turned to 3GPP Release 19, which is scheduled for release in December 2025. The forthcoming update will solve the present interoperability issues between the satellite and ground networks and further enhance NTN capabilities.
Additionally, in underdeveloped markets, end users continue to face obstacles related to pricing and availability. Although the cost of satellite terminals is decreasing, it will still take a few years before they are affordable globally. AI-orchestrated traffic, more software-defined architecture, and international cooperation on standards and space governance are the ways of the future. Overcoming these obstacles will determine the next stage of inclusive, scalable, and resilient global connectivity, both on Earth and in space.
Final Thoughts: Reimagining the Connected Future
Space technology is evolving at a rapid pace, so the next decade is going to be an exciting chapter of breakthroughs—from scalable non-terrestrial networks and intelligent satellite constellations to real-time Earth observation and autonomous in-orbit operations. Want to learn more about satellite trends shaping the future of space communications? Contact Avenga—our extensive expertise in satellite technology is based on cutting-edge device development and product engineering, which enables us to create scalable, intelligent solutions that will drive non-terrestrial connections in the future.